Elly Tanaka is an Adjunct Investigator at the IMP and Scientific Director of the Institute of Molecular Biotechnology of the Austrian Academy of Sciences (IMBA). You find more detailed information about her work and research group at: https://www.oeaw.ac.at/imba/groups/elly-tanaka
Molecular mechanisms of vertebrate regeneration
Unravelling the molecular cell biology of limb and spinal cord regeneration and the evolution of regeneration
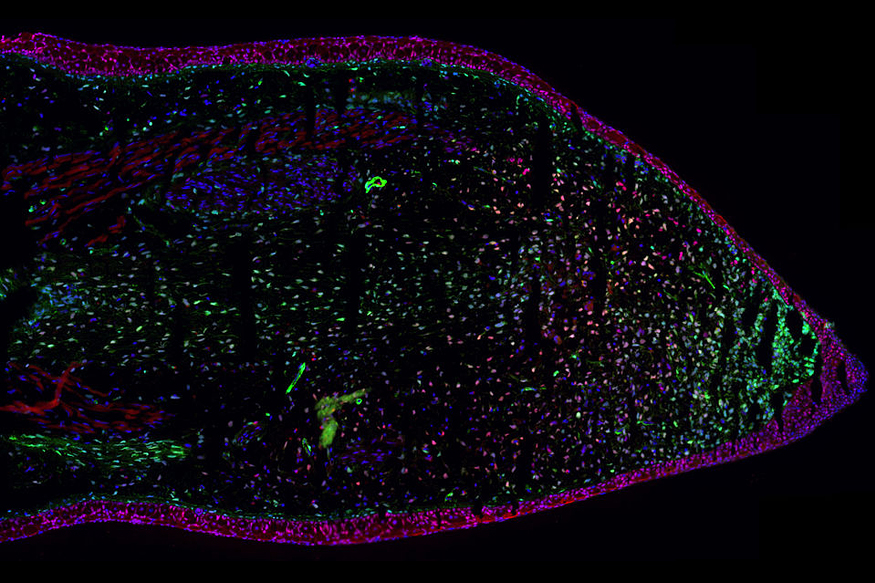
Salamanders have the remarkable capability to regenerate their limbs and spinal cords. Our group has developed molecular genetics in the axolotl in order to identify the stem cells responsible for this complex regeneration, and the injury-responsive signals that initiate their proliferation. We have also identified signals that guide the regeneration process to form a faithfully constructed organ with the right types of cells at the right time. For example, we have recently identified a factor that is released from the epidermis that first forms after an injury.
In addition, we found that, in the salamander spinal cord, adult type neural stem cells dedifferentiate to an embryonic type neural stem cell in order to grow the new spinal cord. This process involves the upregulation of molecular factors involved in planar cell polarity signalling that ensure that the neural stem cells not only divide in the correct orientation, but also cause them to self-renew at the early stages of regeneration. Finally, we are interested in the role of novel gene function in these processes.
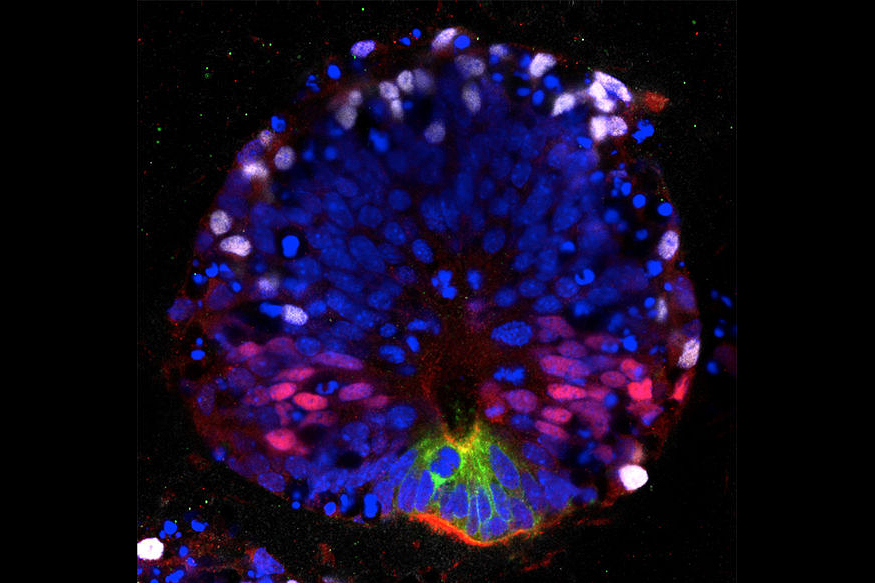
We are now starting to study why the regeneration process ceases to work in frogs after metamorphosis, and why it occurs only in the fingertip of a mouse. We are tracing cognate stem cell populations in these different species to determine if stem cell behaviour and potential vary among animals, and correspondingly we are studying how the cells differ in response to regeneration associated signals.
Translation of regeneration lessons to 3D spinal cord organ formation and retinal function in mouse and man
A key feature of successful regeneration is the formation of a tissue that is patterned in three dimensions. Based on this concept, we have engineered fully patterned three-dimensional spinal cord tissue from mouse embryonic stem cells, and retinal tissue from human embryonic stem cells. Interestingly, global addition of retinoic acid to the mouse spinal cord organoid system causes a spontaneous self-patterning event to give rise to the different neuronal cell types in the right spatial order.
We are actively studying how retinoic acid can confirm this self-organizational ability to the spinal cord organoids. In contrast, in the human system, we have directed human embryonic stem cells to form retinal tissues including the pigmented retinal epithelium (RPE). We are currently using these cells to screen for potential drugs that could ameliorate defects in RPE cells that are known to cause progressive blindness.
Regeneration in Axolotl (Schering Prize 2017)
Selected Publications
- Lust, K., Maynard, A., Gomes, T., Fleck, JS., Camp, JG., Tanaka, EM., Treutlein, B. (2022) Single-cell analyses of axolotl telencephalon organization, neurogenesis, and regeneration. Science. 377(6610):eabp9262
- Lin, TY., Gerber, T., Taniguchi-Sugiura, Y., Murawala, P., Hermann, S., Grosser, L., Shibata, E., Treutlein, B., Tanaka, EM. (2021) Fibroblast dedifferentiation as a determinant of successful regeneration. Dev Cell. 56(10):1541-1551.e6
- Gerber, T., Murawala, P., Knapp, D., Masselink, W., Schuez, M., Hermann, S., Gac-Santel, M., Nowoshilow, S., Kageyama, J., Khattak, S., Currie, JD., Camp, JG., Tanaka, EM., Treutlein, B. (2018) Single-cell analysis uncovers convergence of cell identities during axolotl limb Regeneration. Science. 362(6413)
- Nowoshilow, S., Schloissnig, S., Fei, JF., Dahl, A., Pang, AWC., Pippel, M., Winkler, S., Hastie, AR., Young, G., Roscito, JG., Falcon, F., Knapp, D., Powell, S., Cruz, A., Cao, H., Habermann, B., Hiller, M., Tanaka, EM., Myers, EW. (2018) The axolotl genome and the evolution of key tissue formation Regulators. Nature. 554(7690):50-55
- Sugiura, T., Wang, H., Barsacchi, R., Simon, A., Tanaka, EM. (2016). MARCKS-like protein is an initiating molecule in axolotl appendage regeneration. Nature. 531(7593):237-40
Join us
- Master students and Post-docs: Contact Elly Tanaka with a letter of intent detailing why you want to join the lab.
- PhD students: Calls open 1 March and 1 September, apply here:
Vienna BioCenter PhD Program
Grants

Marie Curie ITN: 101073238 Identifying epigenetic regulators of regeneration gene expression