Mechanics and signalling dynamics in embryogenesis
For embryogenesis to be both precise and robust, information must be efficiently transmitted, and fed back, across scales to seamlessly integrate the molecular signals and gene regulatory networks determining embryo patterning with the mechanical forces and geometrical cues organizing morphogenesis. Morphogens (e.g. Wnt, TGF-β, FGF) – a term coined by Alan Turing in his seminal 1952 theory on morphogenetic patterning – are secreted molecules shown to be highly conserved organizers of embryonic development. Recent work further underlined their importance by showing that, in the presence of a restricted set of these developmental cues, cultured pluripotent cells can self-assemble into embryo- or organ-like structures.
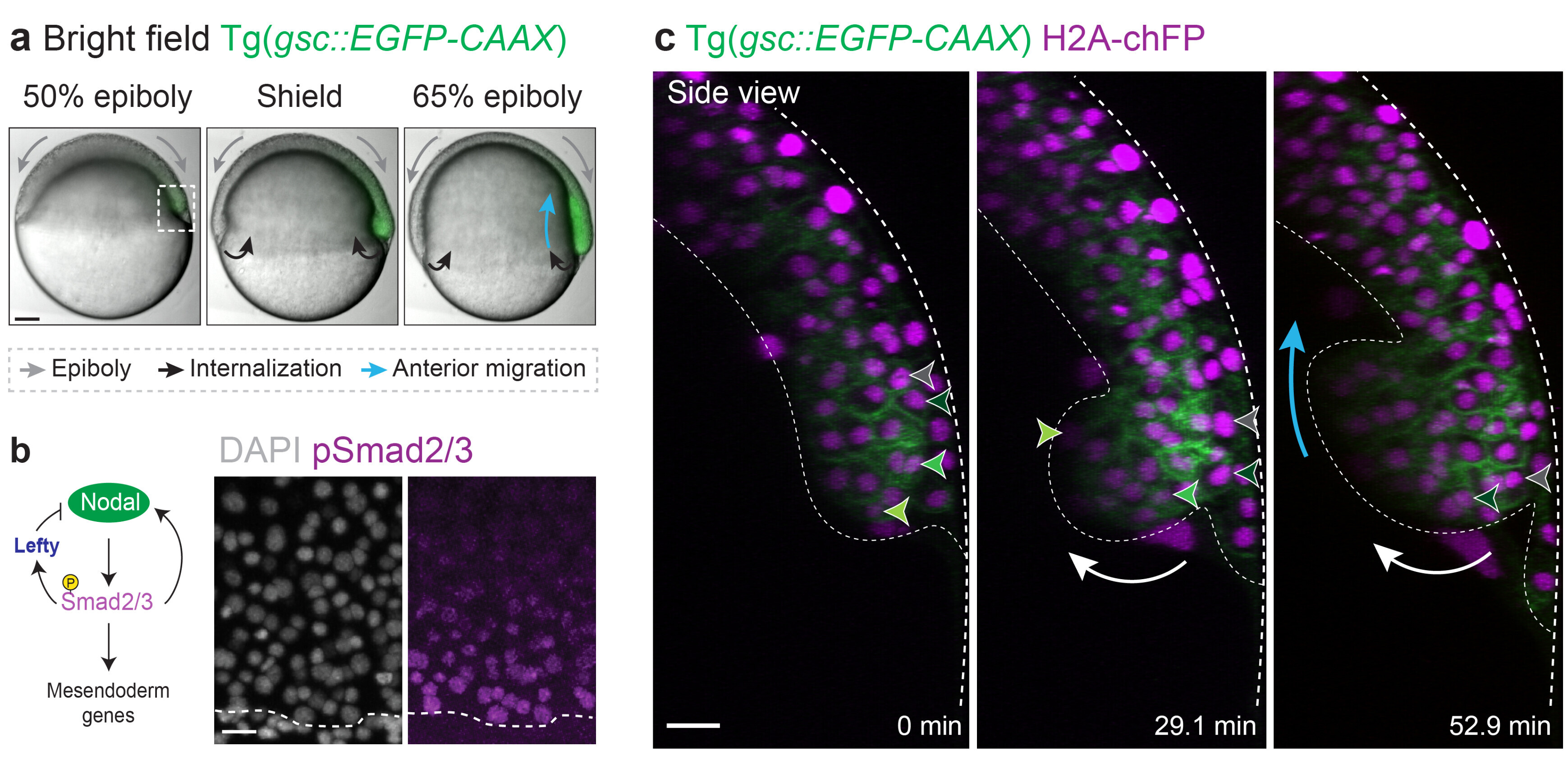
Within developing tissues, morphogens form dynamic spatiotemporal gradients of activity and instruct the transcriptional programs required to produce patterns of discrete cell fates. How cells sense and interpret complex signalling inputs to generate tissue patterning has been under intense scrutiny over the past decade. However, much less is known about how dynamic spatiotemporal features of morphogen signalling (e.g. signalling duration, frequency, combinatorial inputs), encode the mechanical forces, and cellular behaviours, required for embryo morphogenesis. We also need to understand how this morphogenetic information is integrated across scales with emerging tissue patterning.
Morphogen gradient orchestrates pattern-preserving tissue morphogenesis via motility-driven (un)jamming
Our recent work addressed this fundamental question in the context of zebrafish gastrulation, a crucial stage of embryogenesis during which the three germ layers – ectoderm, mesoderm and endoderm – are specified and shaped (Fig. 1a-c). Classical work showed that mesoderm and endoderm (mesendoderm) specification relies on a gradient of the highly conserved Nodal/TGF-β morphogen (Fig. 1b). We showed that Nodal signalling, in addition to its established role in mesendoderm patterning, mechanically subdivides the tissue into leader and follower cells. This arises via a motility-driven (un)jamming transition: whereby cells must overcome a critical value of cell motility forces to drive the cell-cell rearrangements necessary for mesendoderm internalization. Thus, graded Nodal signalling specifies a small fraction of highly protrusive leader cells, able to autonomously internalize via local unjamming, that pull the less protrusive followers to the inside of the embryo (Fig. 2, left panel).
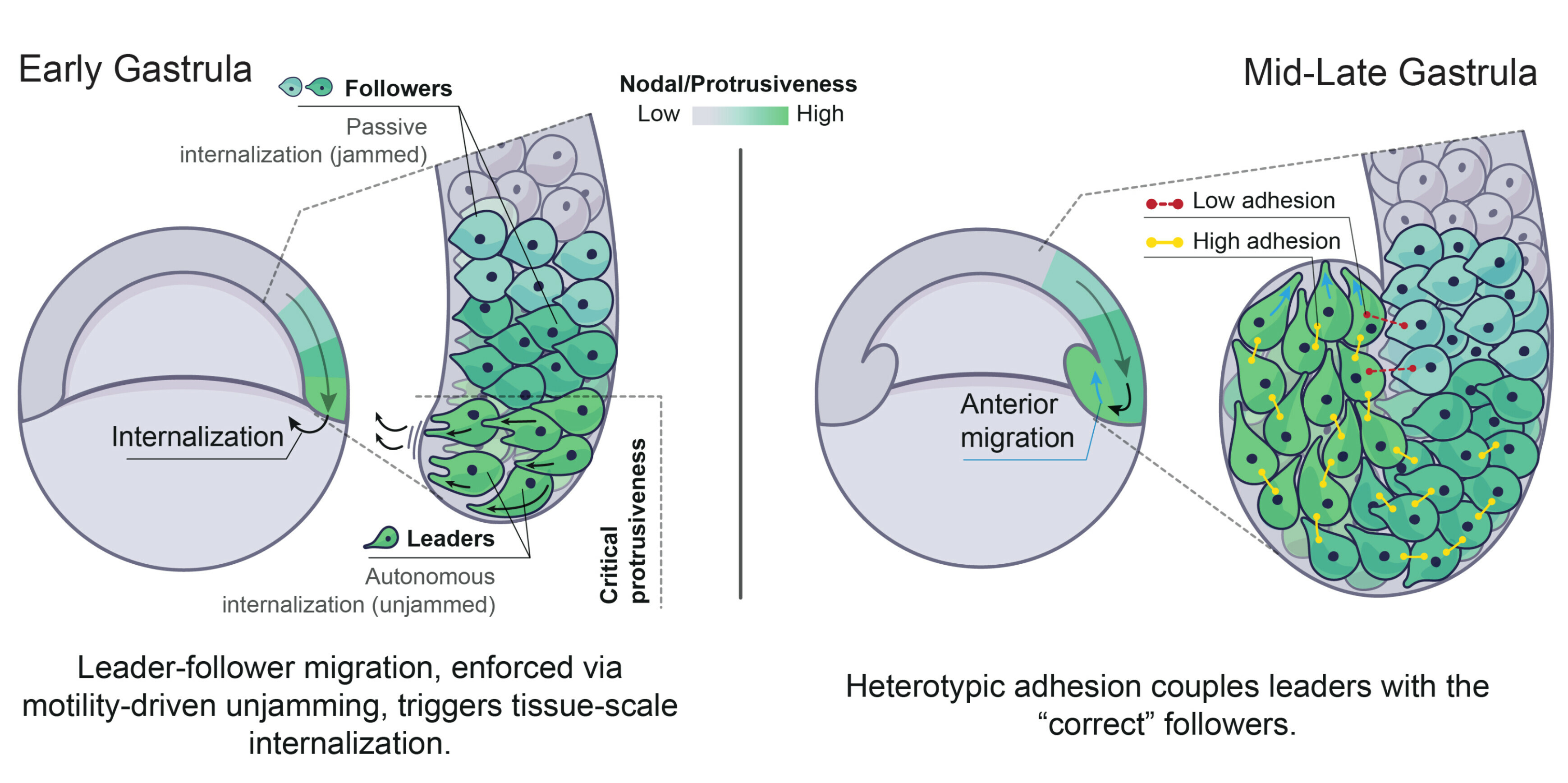
The Nodal signalling gradient further enforces a code of heterotypic, or preferential, adhesion coupling leaders to their immediate followers, resulting in collective and ordered mesendoderm internalization (Fig. 2, right panel). Integrating these distinct mechanical features into minimal active-particle simulations quantitatively predicts both physiological and genetically-perturbed gastrulation movements. Strikingly, this dual mechanical role of Nodal signalling also provides a highly efficient way to ensure that tissue patterning, which is largely completed prior to morphogenesis in zebrafish, is well preserved despite these complex tri-dimensional large-scale movements.
We are particularly interested in the idea that the complex temporal dynamics displayed by single (and multiple) morphogen gradients are key to increase their ability to simultaneously encode diversity of cell fates and mechanical properties. Some key questions in the lab include:
- How do cells integrate dynamic and combinatorial morphogen signalling into well-defined patterns of mechanical behaviours, such as cell migration or adhesion?
- Can we quantify how precise is this signal integration? Could emergent mechanical properties of tissue help to buffer against developmental noise?
- How do mechanical forces and signalling cooperate to give rise to dynamic morphogen gradients? Are there feedbacks?
To answer these questions, we are using a quantitative and live imaging-based approach to measure and perturb both morphogen signalling and mechanical forces. We use the zebrafish embryo as a powerful in vivo system amenable for high resolution imaging and biophysical manipulations, as well human embryonic stem cell colonies (2D gastruloids), which were recently shown to display complex self-organized patterning under minimal culture conditions.
Selected publications
- Pinheiro, D., Kardos, R., Hannezo, É., & Heisenberg, C. P. (2022). Morphogen gradient orchestrates pattern-preserving tissue morphogenesis via motility-driven (un)jamming. Nature Physics, 18, 1482-1493.
- Schauer, A.*, Pinheiro, D.*, Hauschild, R., & Heisenberg, C. P. (2020). Zebrafish embryonic explants undergo genetically encoded self-assembly. Elife, 9.
- Pinheiro, D., & Bellaiche, Y. (2018). Mechanical force-driven adherens junction remodeling and epithelial dynamics. Developmental cell, 47(1), 3-19.
- Pinheiro, D., Hannezo, É., Herszterg, S., Bosveld, F., Gaugue, I., Balakireva, M., ... & Bellaïche, Y. (2017). Transmission of cytokinesis forces via E-cadherin dilution and actomyosin flows. Nature, 545(7652), 103-107.
Join us
- Master students and Post-docs: Contact Diana Pinheiro with a letter of intent detailing why you want to join the lab.
- PhD students: Calls open 1 March and 1 September, apply here:
Vienna BioCenter PhD Program